Highlights
Extracellular nucleotides are key actors of cell signalling through P2 receptors
Dysregulated vesicle release is implicated in immune diseases and cancer
P2 receptors emerge as a mechanism controlling micro- and nano-vesicle release
Abstract
Cell-to-cell communication is a major process for accommodating cell functioning to changes in the environments and to preserve tissue and organism homeostasis. It is achieved by different mechanisms characterized by the origin of the message, the molecular nature of the messenger, its speed of action and its reach. Purinergic signalling is a powerful mechanism initiated by extracellular nucleotides, such as ATP, acting on plasma membrane purinoreceptors. Purinergic signalling is tightly controlled in time and space by the action of ectonucleotidases. Recent studies have highlighted the critical role of purinergic signalling in controlling the generation, release and fate of extracellular vesicles and, in this way, mediating long-distance responses. Most of these discoveries have been made in immune and cancer cells. This review is aimed at establishing the current knowledge on the way which purinoreceptors control extracellular vesicle-mediated communications and consequences for recipient cells.
1. Purinergic signalling and cell communication
The concept of purinergic signalling was proposed by Geoffrey Burnstock, more than fifty years ago and was demonstrated to be responsible to what was initially called the “non-adrenergic, non-cholinergic nerve stimulation” 1, 2. While this signalling pathway initially faced strong scepticism, it is nowadays a fully established cell-to-cell communication pathway recognized for its strong significance, not only in the peripheral nervous system, in both physiological and pathological conditions. Indeed, nucleotides, most importantly adenosine 5'-triphosphate (ATP), but also adenosine 5'-diphosphate (ADP), uridine 5'-triphosphate (UTP), uridine 5'-diphosphate (UDP) and UDP-glucose, are released to the extracellular space in response to various stimuli such as mechanical pressure or distortion, electrical stimulation, osmotic swelling, hypoxia and ischemia, cytotoxic attacks and cell disruption 3, 4, 5. These nucleotides could also be released following cell injury or through non-cytolytic pathways, such as exocytosis or even through plasma membrane channels or transporters 6. Nucleotides, notably ATP, are ubiquitous extracellular signalling molecules, binding to and activating membrane-spanning receptors of the P2 family 5. All mammalian cells express a panel of different P2 receptors having a critical role in physiology and basal tissue homeostasis by modulating essential cellular parameters such as cell proliferation and apoptosis, cell differentiation, migration or invasiveness. In contrast, dysregulation of this signalling pathway contributes to the pathogenesis of various diseases, including inflammatory, neurodevelopmental, neurodegenerative, behavioural and cancerous diseases 7, 8, 9, 10, 11, 12.
P2 receptors are categorized into two distinctive subfamilies in mammalians: ATP-gated ion channel P2X receptors, for which seven genes (P2RX1-P2RX7) encode seven corresponding P2X proteins (P2X1-P2X7) 13, and G-protein-coupled P2Y receptors, for which eight different genes (P2RY1, P2RY2, P2RY4, P2RY6, P2RY11-P2RY14) encode for P2Y proteins (P2Y1, P2Y2, P2Y4, P2Y6, P2Y11-P2Y14) 1. P2X receptors are made of three P2X proteins subunits that can be of the same type (homotrimers) or composed of two different types of subunits (heterotrimers). P2X receptors all function as ATP-gated cationic non-selective channels, permeating K+, Na+ and Ca2+ and showing varied sensitivities to extracellular ATP (eATP) and different rates of desensitization following activation 14, 15. As a result, activation of the P2X receptors causes membrane depolarization and elevates the intracellular concentration of Ca2+ 16, which in turn activates additional transduction pathways such as mitogen-activated protein kinases (MAPK) and Ca2+-calmodulin-dependent enzymes.
Among all P2X receptors, the P2X7 receptor has attracted considerable attention regarding its structural and functional specificities, such as a molecular size higher than all other P2X receptors due a long intracellular carboxy-terminus end allowing specific protein-protein interactions, its unique homo-trimeric assembly and its strikingly low sensitivity to eATP. Indeed, while other P2X receptors are activated with micromolar concentrations of eATP, the activation of the P2X7 receptor (initially called cytolytic P2Z receptor 17) requires hundreds or thousands of micromolar eATP, a condition that arising in few situations such as cytotoxic cell death 18. The activity of the receptor is also characterized by its facilitation process and its ability to induce the formation of large pores across the plasma membrane that allow the passage of organic cations up to 900-1000 Da initially associated with cell death 17, 19, 20, 21. The P2X7 receptor is expressed in a variety of tissues and cells, but is most extensively studied for its crucial role in mediating immune and inflammatory responses and, when dysregulated, in participating in numerous inflammatory diseases 22, 23. The decrease in intracellular K+ concentration caused by the P2X7 receptor activation is a strong stimulus for activation of the NLRP3 inflammasome and the subsequent maturation and release of the potent pro-inflammatory cytokines, interleukin (IL)-1β and IL-18 24. Of interest to this review is the initial hypothesis that P2X7 receptor activation was responsible for release of IL-1β through microvesicle shedding and budding 25, 26, 27, 28.
P2Y receptors are G-protein coupled metabotropic receptors comprised of eight members in humans. Since several different nucleotides are possible agonists for P2Y receptors, they can be subclassified according to their preferred activating ligands as follows: the ATP-selective receptor (P2Y11), the ADP-preferring receptors (P2Y1, P2Y12 and P2Y13), the UTP-preferring receptor (P2Y4), the UTP- and ATP-preferring receptor (P2Y2) and the UDP-glucose or UDP-galactose-preferring receptor (P2Y14). Nevertheless, high concentrations of ATP can also activate P2Y1 and P2Y13 receptors, or inhibit P2Y4 and P2Y12 receptors.
Associated intracellular signalling pathways mostly depend on the type of G-protein these receptors are coupled to. P2Y1, P2Y2, P2Y4 and P2Y6 receptors mainly activate the Gq/11 protein, thus inducing phospholipase C-β (PLC-β)-dependent generation of intracellular second messengers, inositol 1,4,5-triphosphate (IP3) and diacylglycerol, which activate the IP3 receptors and release of endoplasmic reticulum Ca2+ to increase intracellular Ca2+ and protein kinase C (PKC), respectively. The P2Y11 receptor is coupled to the Gq-protein, triggering an increase in intracellular Ca2+ level through an IP3 receptor-mediated release of Ca2+ from the endoplasmic reticulum, or alternatively the Gs-protein, activating adenylyl cyclase (AC) to generate cyclic adenosine monophosphate (cAMP). By contrast, P2Y12, P2Y13 and P2Y14 receptors are mainly coupled to the Gi-protein, thus inhibiting AC and lowering the intracellular cAMP concentration 29. Apart from these conventional pathways, recent studies have described the activation of additional intracellular signalling pathways activated by P2Y receptors, through the recruitment of Gβɣ subunits, such as phosphatidylinositol-4,5-biphosphate 3-kinase ɣ (PI3K-ɣ), phospholipase C-β2 and –β3, G protein-coupled receptor (GPCR) kinase 2 and 3, Rho GTPases and MAPK 30.
The purinergic signalling pathway is delicately and finely regulated in space and time. Indeed, nucleotides show relatively short half-life in the extracellular compartment. This is principally due to their degradation by different types of enzymes, called ectonucleotidases, which are either soluble or attached to the cell surface. These ectonucleotidases hydrolyse nucleotides tri-, di-, and mono-phosphates into their respective nucleosides, with some specific selectivity on substrates. There are four major classes of ectonucleotidases: 1) the ectonucleoside triphosphate diphosphohydrolases (ENTPDases, also referred as Ecto-Apyrases, NTPases or E-ATPases) including CD39, CD39L1 and CD39L3. ENTPDases also hydrolyse dinucleoside polyphosphates, ADP ribose and NAD+, but with a low affinity; 2) the ecto-5′-nucleotidase (5’-NT), also known as CD73; 3) the ectonucleotide pyrophosphatases/phosphodiesterases (ENPPS); and 4) the alkaline phosphatases (APs) 31. The soluble ENTPDase 1, also known as CD39, preferentially hydrolyses ATP into ADP then in AMP. AMP is further hydrolysed to adenosine by the membrane-bound CD73. This is the major source for the production of extracellular adenosine from AMP. CD73 can also hydrolyse other nucleotides mono-phosphates, such as UMP, CMP, GMP and IMP but with a lower affinity than for AMP. While there is no identified receptor activated by extracellular AMP, adenosine is a powerful ligand acting on membrane receptors (originally called P1 receptors) comprising four types of G protein-coupled receptors: A1, A2A, A2B and A3. The activation of these receptors by adenosine, triggers AC stimulation (A2A, A2B) or inhibition (A1, A3), thus increasing or decreasing intracellular cAMP concentration, respectively. Eventually, adenosine is further degraded to inosine by adenosine deaminase (ADA) 32, 33. These findings highlight the high plasticity of nucleotide-stimulated intracellular signalling, depending on the expression of multiple receptors and ectonucleotidases by cells, concentrations and half-life of ligands, activating successively or concomitantly multiple pathways responsible of potentially opposite responses. Considering the rapid degradation of extracellular nucleotides and adenosine, their action is limited in space and time duration.
Recent studies provide evidence to suggest that purinoreceptors are not only involved in autocrine or paracrine cell communication, for a short period of time, but may also be involved in long distances cell-to-cell communications and for a prolonged duration through the release of extracellular vesicles (EVs). While these findings originally came from studies performed in cancer and immune cells, in (Table I), it is most likely that such mechanisms are generalizable to other cell types, in physiological or pathological conditions.
Read more for free (Open Access):
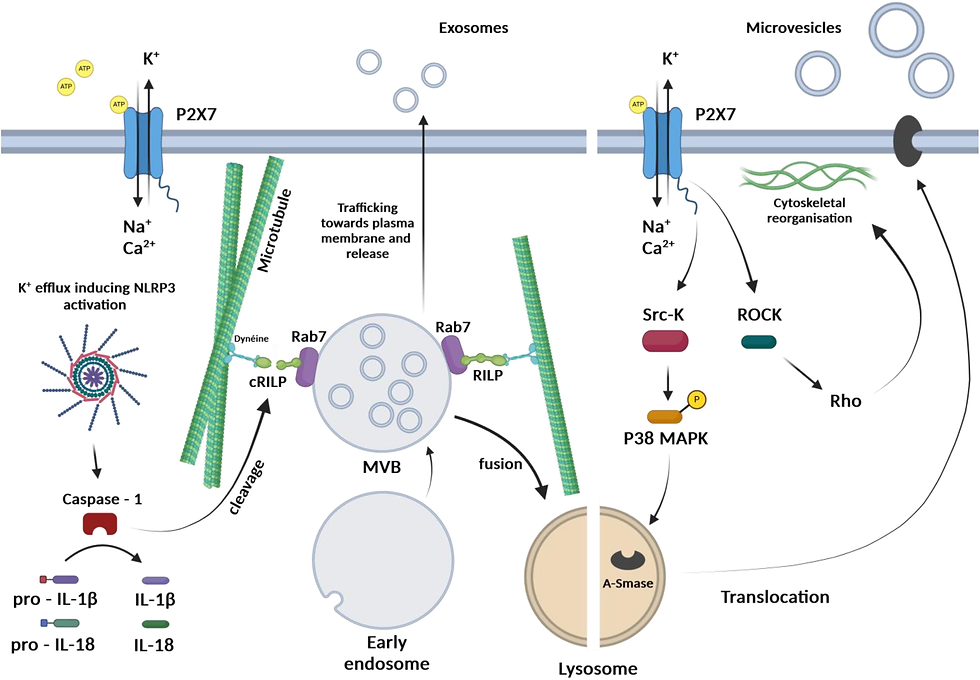
Yorumlar